B.2 - SL Proteins and Enzymes
AMINO ACIDS AND PROTEINS
Proteins are polymers of 2-amino acids, joined by amide links (also known as peptide bonds).
WHAT ARE PROTEINS?
Proteins make up all human tissues like muscles, skin, hair, fingernails and more less obvious things like enzymes. Proteins are made from amino acids which react in a condensation reaction and bond together through peptide bonds to form protein chains.
WHAT’S THE CHEMISTRY?
All amino acids have a common structure, they have a carboxylic acid group, and an amine group. The ‘R’ group which you’re hopefully familiar with from organic chemistry, determines which amino acid it is. There are 20 different naturally occurring amino acids, the simplest of which is glycine where the R group is just H. This R group be anything though.
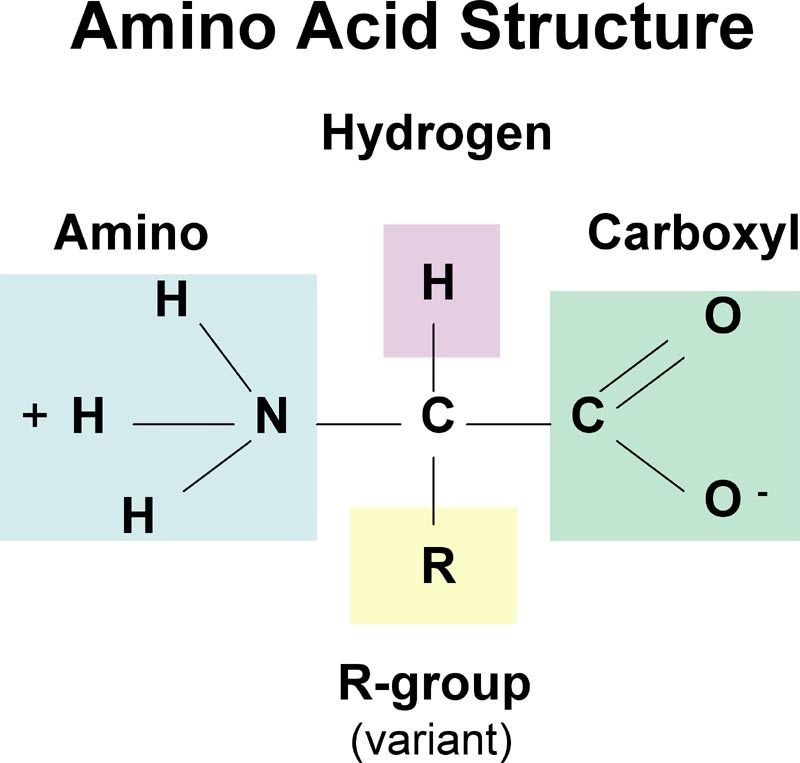
This diagram will be discussed more below (zwitterions), don’t worry if it’s confusing
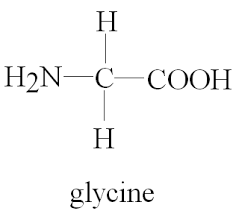
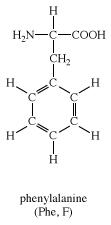
This R group vastly affects the properties of the amino acid.
ZWITTER IONS
Amino acids are amphoteric because of the general structure discussed above. All amino acids have a carboxylic acid group (acidic) and an amine group (basic). This makes amino acids amphoteric because they can react with both acids and bases.
LOW PH
In a low pH situation, the amine group reacts with H+ to form a cation.

HIGH PH
At a high pH, the carboxylic acid group reacts with OH- to form an anion.
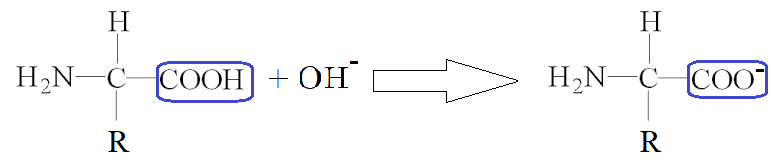
ZWITTER IONS
Zwitterions (Zwitter ions) are when a molecule is both an anion and a cation at the same time. This can occur with amino acids. At a certain pH, amino acids have both a H3N+ group, and a COO- group.
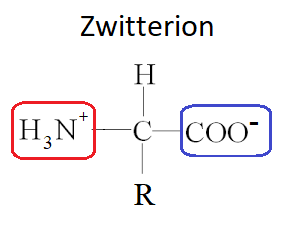
EFFECT OF THE R GROUP ON AMINO ACID PROPERTIES
Explanation of the solubilities and melting points of amino acids in terms of zwitterions.
pI - ISOELECTRIC POINT
The pH at which this zwitterion occurs is called the Isoelectric point (pI). Every amino acid has it’s own isoelectric point, which is dependant on the R group. An acidic R group leads to a lower pI and a basic R group leads to a higher pI.

An extra COOH group in the R group leads to a low pI

Only a H for the R group leads to a pI of 6

Several NH and NH2 groups in the R group leads to a high pI
At a pH higher than that of the isoelectric point, a cation will form and at a pH lower than the isoelectric point, an anion will form.
MELTING POINT
As you will have learnt in topic 4, melting points are dependant on Intermolecular Forces (IMFs). An R group with a large amount of intermolecular forces will cause the amino acid to have a high melting point. This syllabus statement is basically a repeat of what has already been learnt in topic 4.
SOLUBILITY
Same as above. A more polar R group will be more soluble, just like you learnt in topic 4.
PROTEIN STRUCTURES
PRIMARY STRUCTURE
This is a protein’s one dimensional structure. This is just looking at the sequence of amino acids that are joined to each other.
SECONDARY STRUCTURE
This is looking at proteins on a broader level. Protein chains can fold or coil depending on intramolecular forces in the protein chain. There are two types of secondary structures that proteins can form (besides straight chain).
ALPHA HELIX
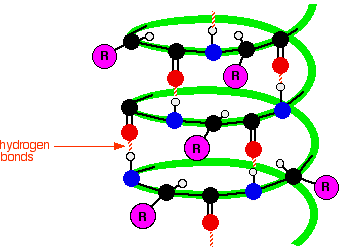
Basically a cool looking spiral shape. This is caused by intermolecular forces between different sections of 1 chain that forces the protein into a helix shape. It’s usually drawn as a ribbon so that it’s helix structure is more easily seen.
BETA PLEATED SHEET
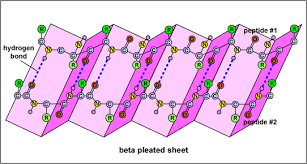
The beta pleated sheet occurs when 2 or more chains (or parts of chains) ‘line up’ due to intermolecular forces and form a sheet
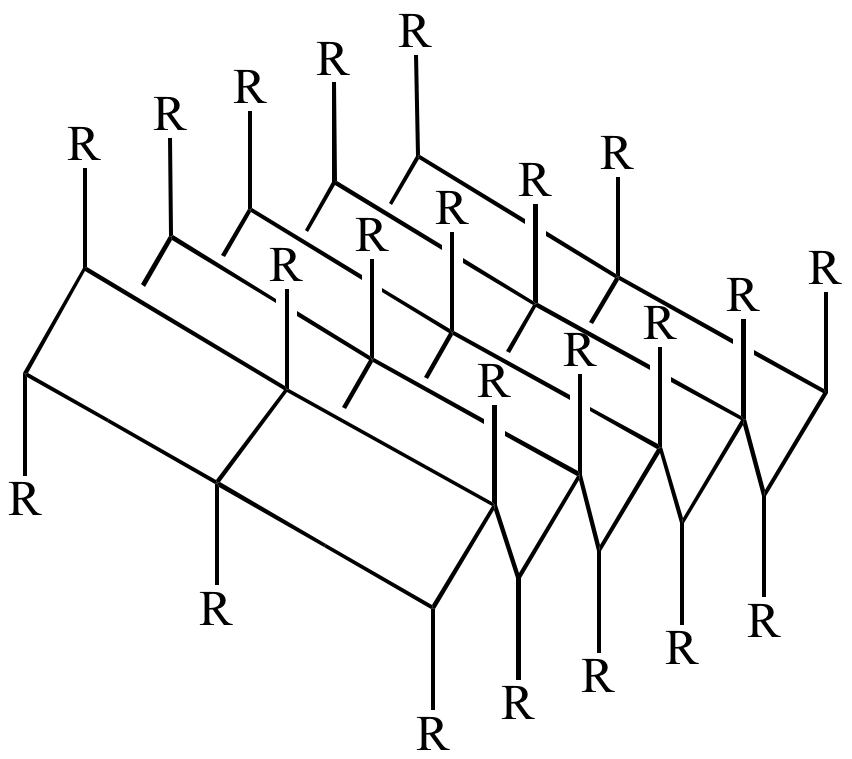
TERTIARY STRUCTURE
This is an even broader look at a protein. In 1 protein, there’s likely to be sections that have an alpha helix structure, and some sections with a beta sheet structure. Looking at the protein chain as a whole is looking at it’s tertiary structure.
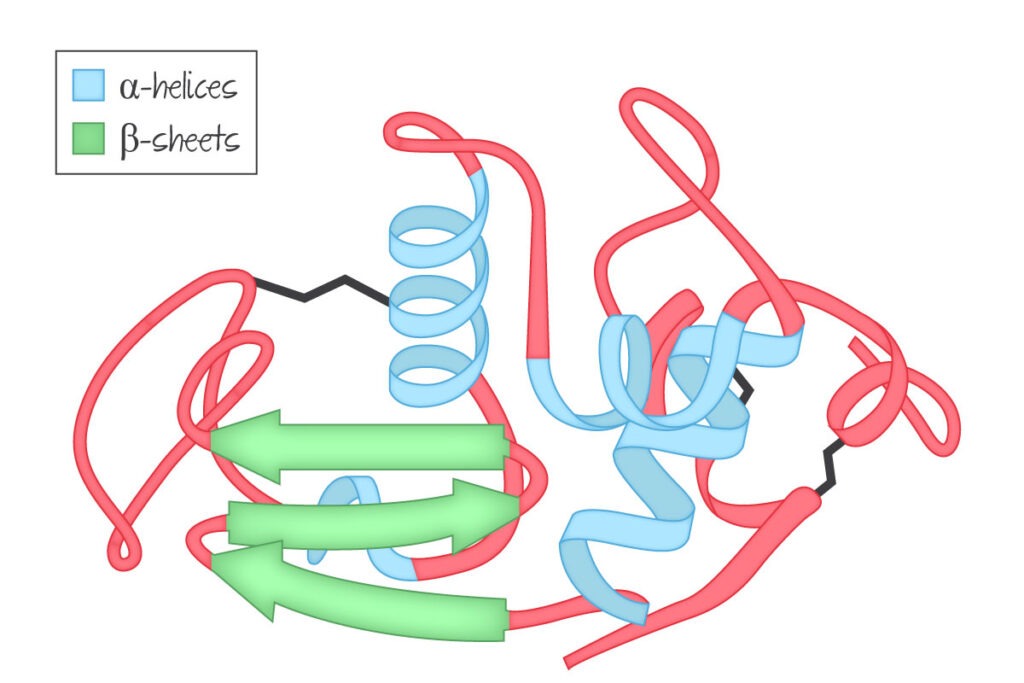
This diagram shows the tertiary structure of this protein, which has a mixture of alpha helices and beta sheets.
QUATERNARY STRUCTURE
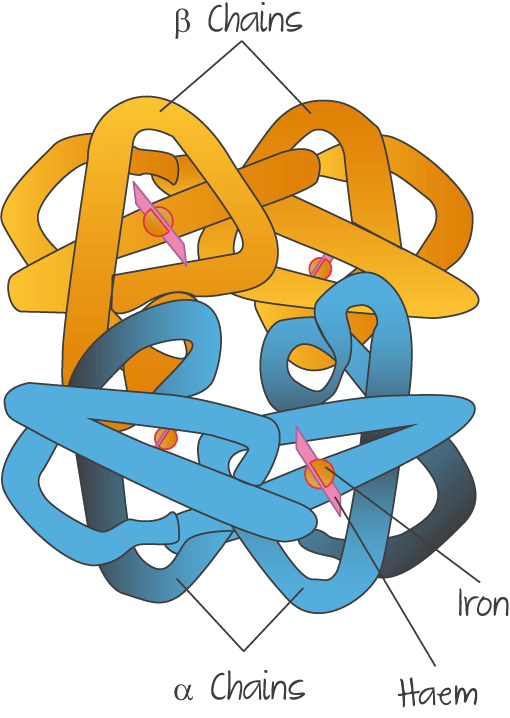
An again broader view of proteins. The tertiary structure looked at only one protein chain. The quaternary structure could have multiple chains bonded together. A common example of this is haemoglobin, which has 4 protein chains bonded together.
EFFECT OF SHAPE ON FUNCTION
A protein’s three-dimensional shape determines its role in structural components or in metabolic processes.
GLOBULAR PROTEINS
Haemoglobin is an example of a globular protein. Globular proteins usually have complex tertiary and quaternary structures, and are usually somewhat spherical. Because of their complex structure, they are more heat sensitive. The protein chain in a globular protein usually has it’s polar R groups exposed, and is therefore more soluble in water.
FIBROUS PROTEINS
Fibrous proteins have little or no tertiary or quaternary structures at all, and form long fibres. This structure makes them much ‘stronger’ than globular proteins. Their polar R groups are not exposed like in globular proteins, making them insoluble. Examples of fibrous proteins would be in hair, skin and bones, where insolubility and structure are needed.
ENZYME BASICS
Most enzymes are proteins that act as catalysts by binding specifically to a substrate at the active site.
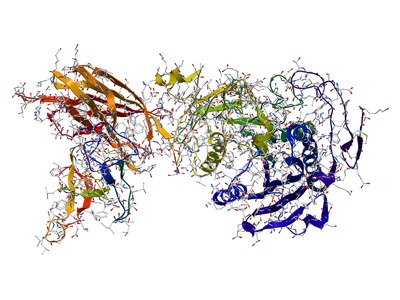
An example of an enzyme
Enzymes are biological catalysts that speed up reactions within the body. Enzymes are usually complex proteins.
Some examples of enzymes are:
- Amylase
- Protease
- Maltose
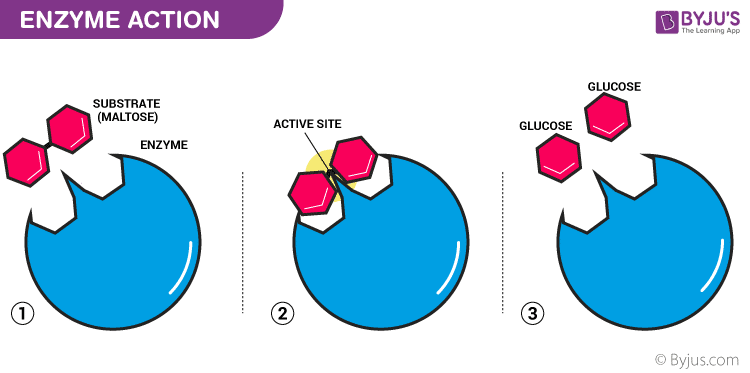
A simple diagram of the mechanism of an enzyme catalysed reaction. More on this later…
ENZYME MODELS
LOCK AND KEY
The lock and key model is the most basic model of enzymes, that is shown above.
- The enzyme is designed to work for only one substrate, for which the active site is specifically 'moulded'. The substrate is therefore a perfect fit for the active site
INDUCED FIT MODEL
The induced fit model suggests that the enzyme plays a role in forming the shape of the active site. The intermolecular forces from the substrate therefore help mould the shape of the active site so that the substrate fits.
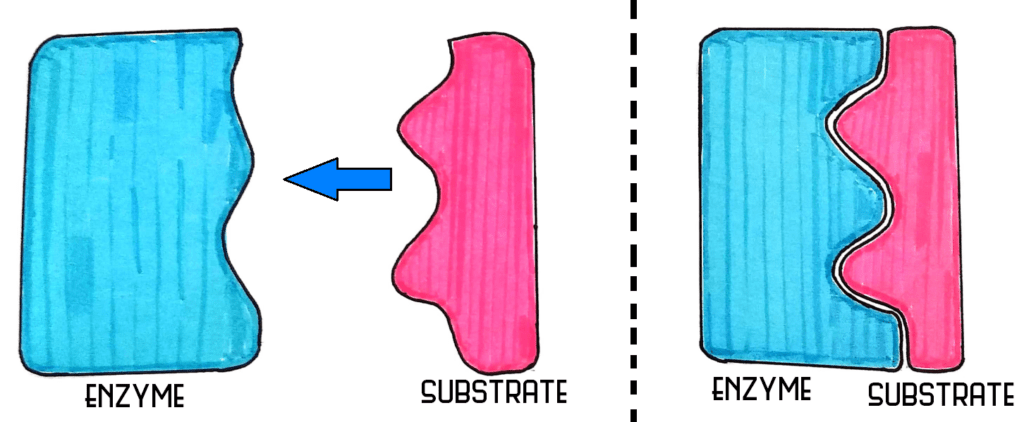
ENZYME ACTIVITY
As enzyme activity depends on the conformation, it is sensitive to changes in temperature and pH and the presence of heavy metal ions.
EFFECTS OF PH AND TEMPERATURE ON ENZYMES
pH and temperature both have the effect of denaturing the enzyme. Denaturing just means that the active site has been damaged and can no longer function.
Enzymes all have their own ranges of temperature and pH that they work at. For most enzymes, this is the temperature and pH of most organisms.
Each enzyme has a different optimum pH and temperature depending on where they operate in the body. Some examples are:
- Amylase (in the mouth)
- pH 6.7
- 32-37 C
- Catalase (in plants)
- pH 7
- 48C
- Protease (in the stomach)
- pH 1.6
- 50C
HEAVY METAL IONS
Heavy metal ions act as non-competitive inhibitors. This is a HL concept from B.7 so I won’t include the details in this post but the long and the short of it is that it indirectly destroys the active site.
ENZYME ACTIVITY
Deduction and interpretation of graphs of enzyme activity involving changes in substrate concentration, pH and temperature.
This is basically the kinetics of enzymes. The graphs you will come across here are graphs of Rate of Reaction against Substrate concentration. Because an enzyme can only work at a certain maximum rate, the graph curves off and a maximum rate of reaction is seen.
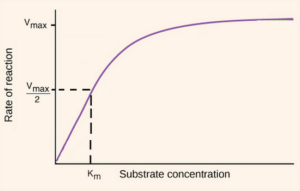
From this maximum, a ‘half max’ can also be calculated a bit like they do for half life in physics (and medicinal chem <3). This ‘half max’ is used to calculate a constant called the Michaelis constant (Km). Every enzyme has a different Michaelis constant.
EFFECT OF PH AND TEMPERATURE (GRAPHS)
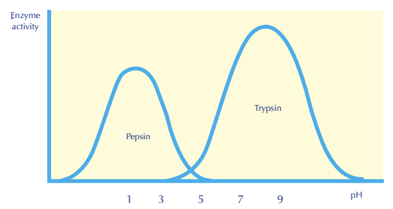
Enzyme activity plotted against pH for 2 different enzymes
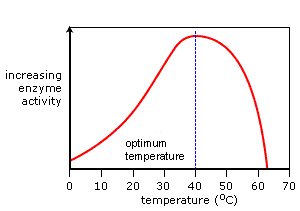
CHROMATOGRAPHY AND ELECTROPHORESIS
CHROMATOGRAPHY
Hopefully you remember this from GCSE. Chromatography is the separation of different substances based on their solubility in a solvent.
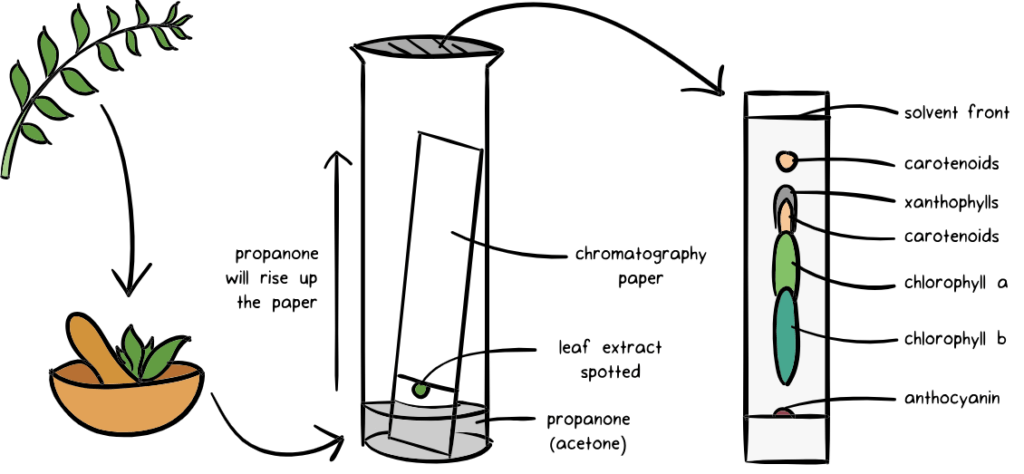
Chromatography also doesn’t necessarily need to be done using paper, it can also be done using a gel 🙂
ENZYME CHROMATOGRAPHY
- A spot of the sample is put in the bottom left corner of the paper and is then placed in the first solvent.
- Once the 1st chromatography is finished, the paper is turned 90 degrees clockwise, and placed in a second solvent.
- The different spots can then be analysed based on their Rf values in both of the solvents.
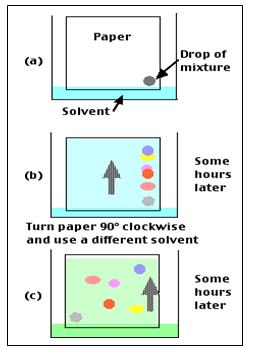
RF VALUES
The Rf value is a ratio of the distance travelled by the solute and the distance moved by the solvent.
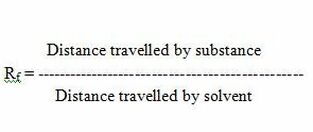
ELECTROPHORESIS
Electrophoresis is another technique that can be used to separate and identify proteins and/or amino acids based on their Isoelectric point (pI). Electrophoresis uses a gel or paper soaked in a buffer solution to separate the amino acids. At the buffer pH (usually 6), some amino acids will form positive or negative ions based on their isoelectric points. These ions are then attracted to the electrodes in the electrophoresis setup.
Electrophoresis can either be done using paper or a semi-firm gel. The samples to be tested are applied in the centre of a piece of paper or in a cavity in the gel. An electric field is applied over the gel or paper. Due to the electric field, the proteins and amino acids will seperate.
METHOD
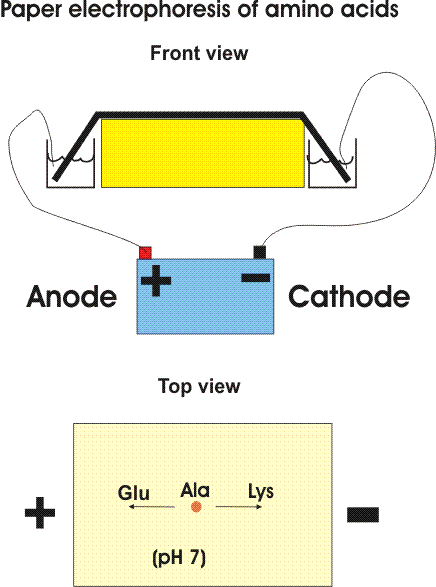
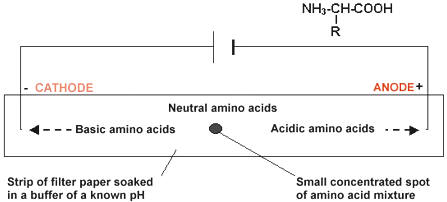
FACTORS AFFECTING SEPARATION
The separation of the amino acids and proteins depends on how they interact with the gel or the paper and solvent. This can be affected by:
- The molecular weight (size) of the protein or amino acids
- A a small, light amino acid like Lysine will interact less than a large protein like an enzyme
- The shape of the protein or amino acid (e.g. tertiary structure)
- This is similar to molecular weight in that a bigger protein will interact more than a small, ‘streamlined’ amino acid.
- The charge/s on a protein or amino acid
- The separation in electrophoresis is based on electric charge and the molecules attraction to the + and – of the electrophoresis setup. If the charge is bigger, the attraction will be bigger and the amino acid or protein will travel further.
NATIVE AND DENATURING ELECTROPHORESIS
NATIVE ELECTROPHORESIS
- Enzymes and proteins are in their natural form
- Separation in electrophoresis is therefore based on their size AND shape
DENATURING ELECTROPHORESIS
- Sample is chemically or thermally denatured
- This is done to break the intramolecular forces ‘within’ the proteins so that they have not tertiary structure. The protein chains are linearized.
- Separation in electrophoresis is therefore based on their size ONLY